The application of high-stability pressure and differential pressure transmitters is becoming increasingly important in the field of automation. Developing high-stability pressure transmitters is a significant challenge that manufacturers face.
However, it has been demonstrated through practice that significant improvements in the overall performance, accuracy, and reliability of high-stability pressure and differential pressure transmitters can be achieved through breakthroughs in various technological aspects.
These aspects include the selection of ultra-stable monocrystalline silicon principle chips, stress-free packaging of ultra-stable monocrystalline silicon wafers, elimination of hysteresis errors, reduction and compensation of static pressure errors, expansion of the range ratio of the instruments, special treatment of the wetted surface, and ultra-high-temperature measurement techniques.
Current High Stability Pressure and Differential Pressure Transmitters
The mainstream high-stability pressure and differential pressure transmitters in the current market are primarily of three types and principles.
1.1 Metal Capacitive Pressure Principles
One type is the metal capacitive pressure and differential pressure transmitters developed and produced by some manufacturers, with representative models such as the 1151, 3051, and 3351 series.
Their working principle is as follows:
- The external pressure difference is transmitted to the internal metal capacitor plates.
- When the plates are displaced, a change in capacitance occurs.
- This change in capacitance is collected, amplified, and processed with software compensation by electronic circuits, resulting in a linear output of the pressure signal.
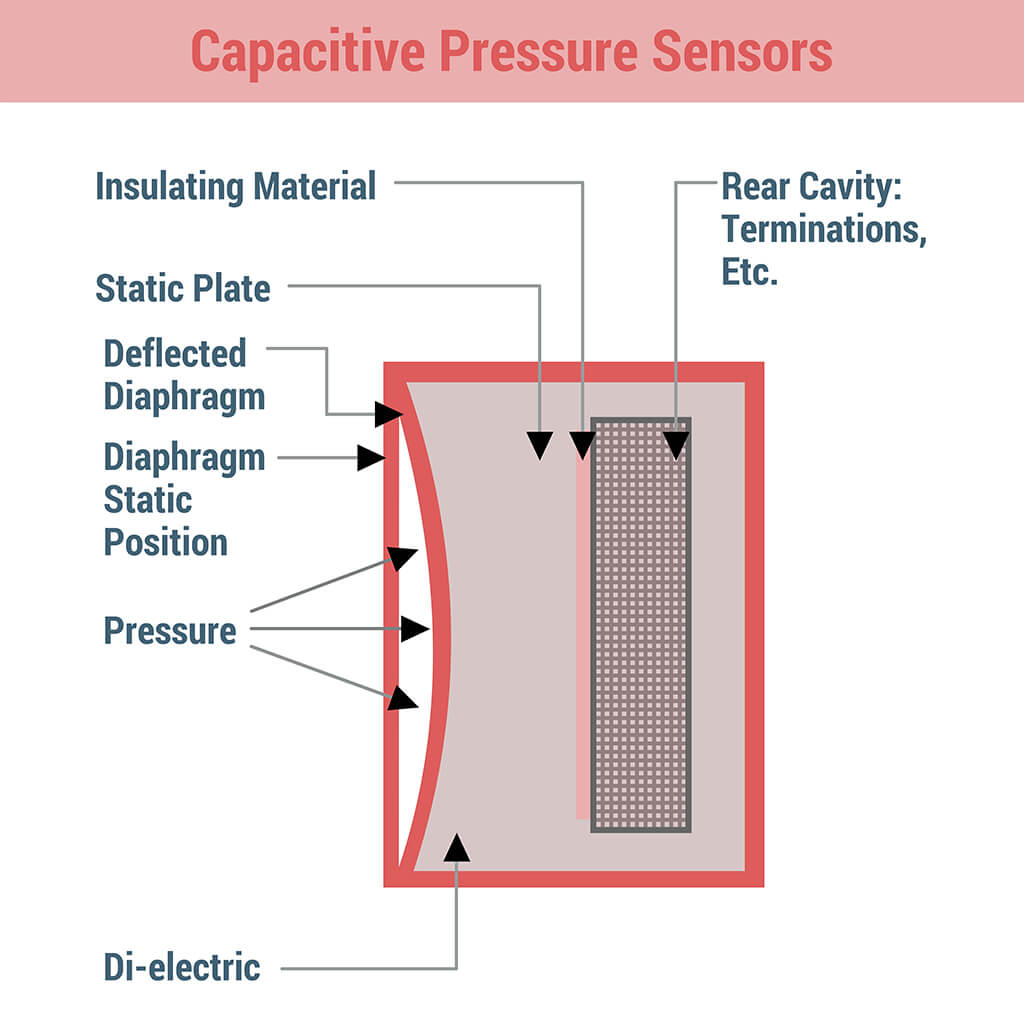
Since the technology of the 1151 series capacitive sensors was introduced to China in the 1980s, it has been widely replicated and promoted domestically.
By 2016, there were nearly 100 local manufacturers, with more typical domestic manufacturers located in Shanghai, Xi’an, Beijing, Chongqing, and other companies under the Nuclear Industry Department.
After years of research and exploration, most of above instrument manufacturers began to miniaturize the 1151 transmitters at the beginning of the 21st century. The volume was significantly reduced, and the transition from analog to digital circuits was gradually made, eventually achieving an improvement in accuracy grade from 0.5 to 0.1.
However, these improvements did not fundamentally change the sensor structure; therefore, despite the advancements, there were still considerable limitations.
The accuracy, long-term stability, EMI performance, static pressure performance, and temperature performance still had a significant gap compared to their original counterpart’s products. This ultimately led to the situation where many pressure transmitters remained far behind those from EU and USA.
However, the 3051C/S series transmitters have made significant improvements based on the 1151 series, achieving substantial enhancements such as structural isolation, suspension, and improved circuit reliability. These transmitters have reached an accuracy level of 0.05%, a significant leap in performance.
However, the technical difficulties and barriers presented by the 3051C/S pressure transmitters have not been effectively overcome by Chinese domestic enterprises, leading almost all Chinese manufacturers to abandon further exploration and research into capacitive transmitters.
In other words, currently, most China domestic manufacturers in the field of metal capacitive pressure transmitters can only maintain an accuracy level of 0.1-0.2%.
If 0.1-0.2% accuracy class can be accepted and workable for your application, please click to check one of leading example produced by Eastsensor: ESS343 (the metal capacitive sensing element and sensor core)
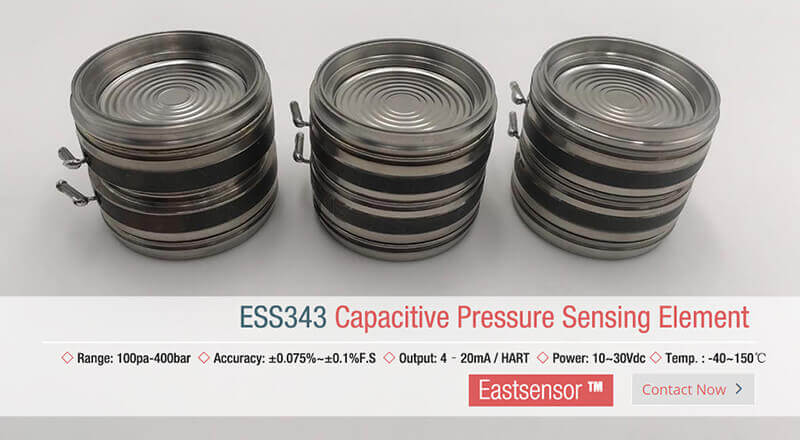
1.2 Monocrystalline Silicon Pressure Principles
The second type is the monocrystalline silicon pressure transmitters and differential pressure transmitters developed and produced by Japanese manufacturers, with representative models being the Yokogawa EJA and EJX series.
Their working principle is as follows:
- The external pressure difference is transmitted to the internal monocrystalline silicon resonant beam.
- Under the action of pressure, the resonant beam generates a pair of differential frequency signals that vary with the pressure.
- These differential frequency signals are then collected, amplified, and processed with software compensation by electronic circuits, resulting in a linear output of the pressure signal.
The Yokogawa EJA series monocrystalline silicon resonant transmitters have certain advantages in manufacturing cost control compared to capacitive sensors.
The main advantages are reflected in the temperature and static pressure compensation stages, that is, the original differential signal output of the dual resonant circuit, which is not affected by temperature and static pressure, making the temperature compensation and static pressure compensation processes of the transmitter relatively simple.
These transmitters ultimately reach an accuracy level of 0.065%, slightly inferior to the Rosemount 3051C/S series.
However, since the bulk production technology of monocrystalline silicon resonant beam chips is monopolized by the company Yokogawa, the technical difficulties and barriers brought by these chips cannot be effectively overcome by Chinese domestic enterprises either.
Therefore, almost all Chinese manufacturers have also abandoned further exploration and research into monocrystalline silicon resonant transmitters.
1.3 Monocrystalline Silicon Resistive Pressure
The third type is represented by German and Swiss monocrystalline silicon resistive pressure and differential pressure transmitters.
Their working principle involves
- An external pressure difference being transmitted to a monocrystalline silicon full-dynamic piezoresistive Wheatstone bridge inside the device.
- The Wheatstone bridge generates a voltage signal that varies with pressure.
- This voltage signal is collected, amplified, and processed with software compensation through electronic circuits to produce a linear pressure signal output.
These monocrystalline silicon resistive sensors are characterized by high output sensitivity, large signal output, and minimal hysteresis. Additionally, their circuit design tends to be simple and reliable.
Therefore, many Chinese domestic transmitter manufacturers prefer this approach for the development and manufacturing of high-end transmitters.
However, compared to metal capacitive sensors and monocrystalline silicon resonant sensors mentioned earlier, the application of monocrystalline silicon resistive chips has specific technical requirements.
These are mainly reflected in the stress-free packaging technology of silicon chips and the unidirectional overload protection technology of silicon thin films. These two application technologies were firmly held by Western countries before the year 2000.
After 2010, Chinese enterprises, through technical cooperation, introduction, and further research and development with some Swiss companies, have fully grasped multiple related technologies.
This has enabled the large-scale domestic manufacturing of high-stability silicon pressure and differential pressure transmitters.
EST4300M Monocrystalline Silicon Pressure Transmitters which designed and manufactured by Eastsensor, is one of them.
The accuracy level of the EST4300M series of high-stability monocrystalline silicon transmitters has reached 0.075%, which is better than 0.1%-0.2% of EST4300, and narrowing the gap with renowned transmitter brands from industrialized countries.
1.4 Working Principle of Monocrystalline Silicon Pressure Transmitters
As shown in below figure, the sensitive element of the EST4300M monocrystalline silicon sensor is created by diffusing P-type impurities onto an N-type silicon wafer, forming a very thin conductive P-type layer.
When leads are soldered on, it becomes a “monocrystalline silicon strain gauge.” Its electrical performance is that of a full-dynamic piezoresistive effect Wheatstone bridge.
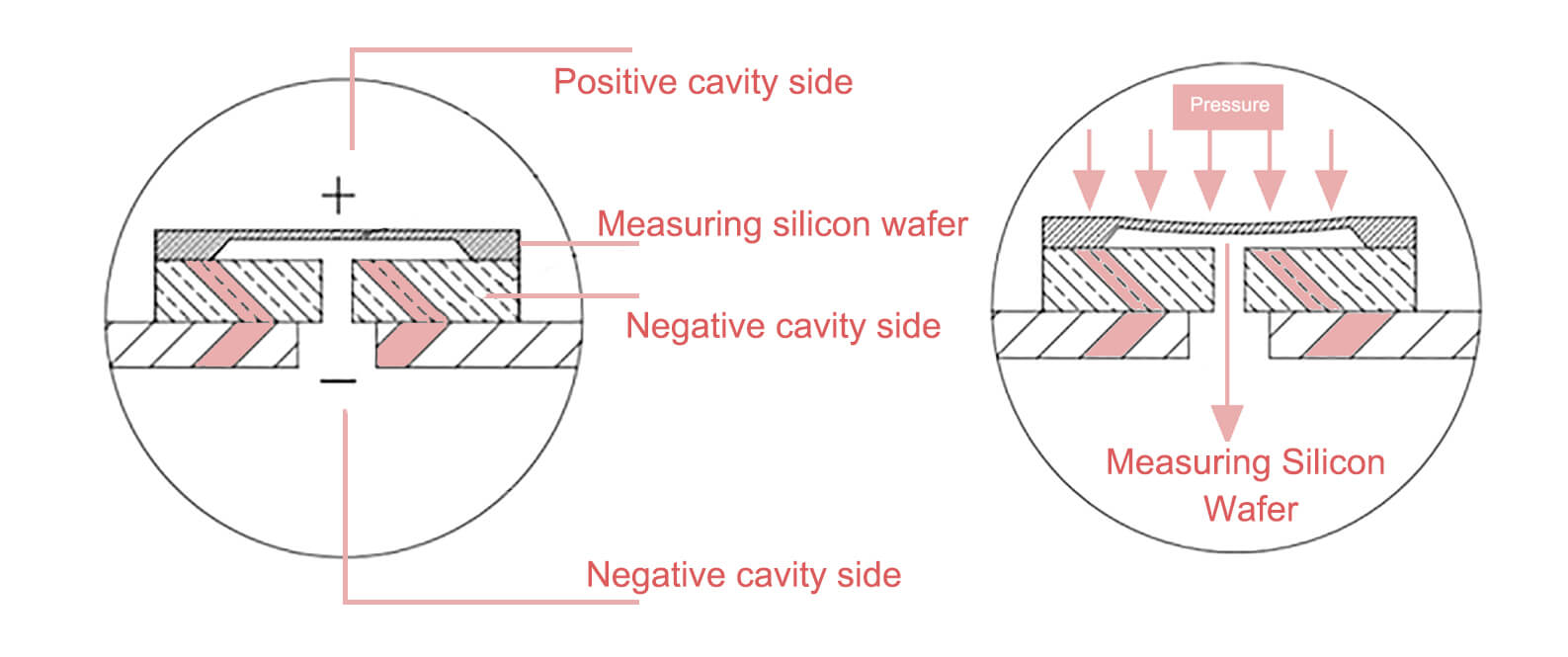
This piezoresistive Wheatstone bridge is combined with elastic elements (the N-type silicon substrate). The medium pressure is transmitted to the positive cavity side of the silicon diaphragm through sealed silicon oil, creating a pressure difference with the medium acting on the negative cavity side.
The combined effect causes one side of the diaphragm to compress and the other to stretch. The pressure difference unbalances the bridge, producing a signal corresponding to the pressure change. After electronic circuit processing, the Wheatstone bridge’s output signal generates a linear 4-20mA standard signal output in relation to pressure changes.
- For gauge pressure sensors, the negative cavity side is usually open to the atmosphere, using atmospheric pressure as the reference pressure.
- For absolute pressure sensors, the negative cavity side is typically a vacuum chamber, using a vacuum as the reference pressure.
- For differential pressure sensors, the negative cavity side’s pressure medium is usually the same as the positive side, such as silicon oil, fluorinated oil, vegetable oil, etc.
As described in above figure, under the effect of the pressure difference between the positive and negative chambers, the measuring silicon diaphragm (also known as the elastic element) bends and deforms.
When the pressure difference P is below the required stress proportionality limit σp of the measuring silicon diaphragm, the bending can be fully reversed.
However, when the pressure difference P exceeds σp, the material reaches its yield stage and even the hardening stage. At this point, if the pressure difference is removed, the measuring silicon diaphragm cannot return to its original position, resulting in irreversible measurement deviations.
If the pressure difference P reaches or exceeds the maximum stress σb that the measuring silicon diaphragm can withstand, it ruptures, causing direct damage to the sensor.
Therefore, by preventing or weakening the direct transmission of the external overload pressure difference P to the measuring silicon diaphragm, one can effectively protect the measurement accuracy and lifespan of the sensor. This leads to the issue of designing overload protection for the monocrystalline silicon chip.
EST4300M- One of the most Reliable Monocrystalline Silicon Pressure Transmitters
EST4300M is type of Monocrystalline Silicon Smart Pressure Transmitter, which designed and manufactured by Eastsensor, it features 4-20mA+Hart to deliver accurate (0.075%/FS) outputs for data collection and control devices.
The precision and stability of EST4300M Monocrystalline Silicon Pressure Transmitter stand as their most distinguishing features, it boasts incredibly high accuracy, typically reaching levels up to 0.075% or 0.1% of Span.
This simply means that the margin of error in the detection and transmission of pressure levels is minuscule. Consequently, tasks that require minute attention to detail and accuracy can be conducted with confidence, knowing these transmitters provide one of the highest performance levels in pressure sensing.
EST4300M has recognized for their stability over extended periods, it has a remarkably low drift rate of less than 0.25% per annum. This characteristic means that EST4300M offer consistent, reliable information about pressure levels that remains accurate over the long term.
This kind of stability also ensures that processes relying on pressure measurement continue operating optimally, without the need for regular sensor recalibrations or replacements.
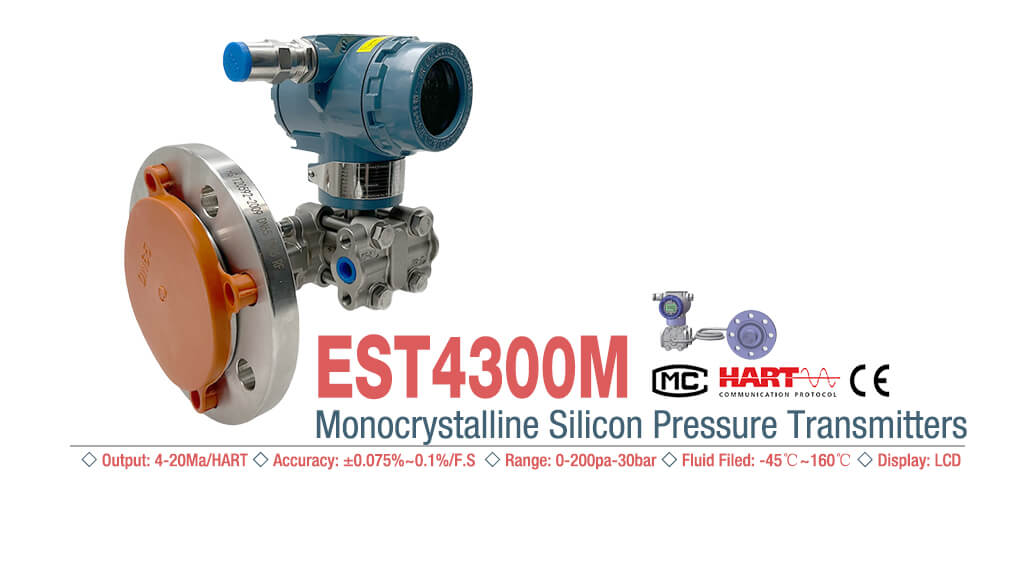
On top of precision and stability, you can also find below details about other features of EST4300M.
2.1 Pressure Overload Protection Design and Implementation
To overcome the deficiency of the poor overload resistance of monocrystalline silicon chips, the EST4300M is equipped with a differential pressure sensor that has unidirectional pressure overload protection.
As shown in below figure, this unidirectional pressure overload protection differential pressure sensor is not only capable of measuring the pressure difference within the rated pressure range in the field conditions but also can effectively protect itself in the event of a unidirectional pressure overload.
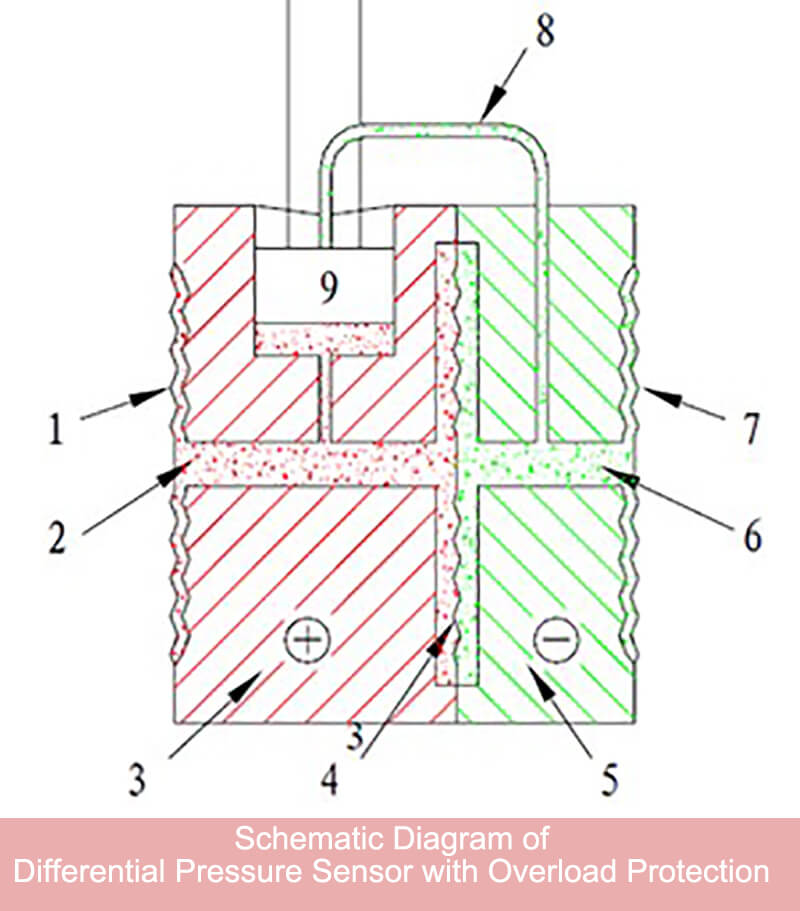
This avoids damage caused by unidirectional pressure overload of the silicon differential pressure sensor.
Such overload protection is typically achieved through design features that limit the deformation of the silicon diaphragm.
For example, mechanical stops may be incorporated into the sensor design, which come into play when a certain deformation threshold is exceeded, thereby preventing further deformation and potential damage to the silicon diaphragm.
Additionally, the material properties and thickness of the diaphragm can be selected to provide the necessary resistance to overload while still allowing the necessary sensitivity for accurate measurement within the normal operating range.
The protection mechanism can be passive, where the design inherently protects the sensor, or active, where electronic or mechanical components react to the overload condition to prevent damage.
In some cases, a combination of both passive and active protection features is used to ensure the sensor’s longevity and reliability.
The description provided outlines a safety mechanism for protecting the monocrystalline silicon chip within a differential pressure sensor from pressure overloads that exceed its allowable working range.
The operational principle of this overload protection can be summarized as follows:
- When a differential pressure that is higher than the allowable working range of the differential pressure measuring silicon diaphragm occurs, a central isolation diaphragm within the sensor moves toward the low-pressure side.
- This movement causes the external isolation diaphragm on the high-pressure side to align with the inner wall of the chamber.
- Consequently, the silicon oil on the high-pressure side is forced entirely into the chamber and cannot transmit any higher pressure to the monocrystalline silicon chip.
- By preventing further pressure transmission, the occurrence of excessively high pressure on the monocrystalline silicon chip is avoided, effectively protecting it from overload.
This mechanism is an example of a passive protection system. In essence, the sensor’s structure is designed in such a way that it naturally adapts to excessive pressure conditions and prevents them from affecting the sensitive measuring component, i.e., the monocrystalline silicon chip.
By using a movable isolation diaphragm and a strategically placed external isolation diaphragm, the system ensures that the pressure is not transmitted beyond a certain threshold, which would otherwise compromise the integrity and accuracy of the pressure sensor.
This design is crucial for applications where pressure sensors are subject to fluctuating or unexpected pressure spikes. It ensures that the sensor can continue to operate within its specified parameters and provides reliability and longevity to the system it is monitoring or controlling.
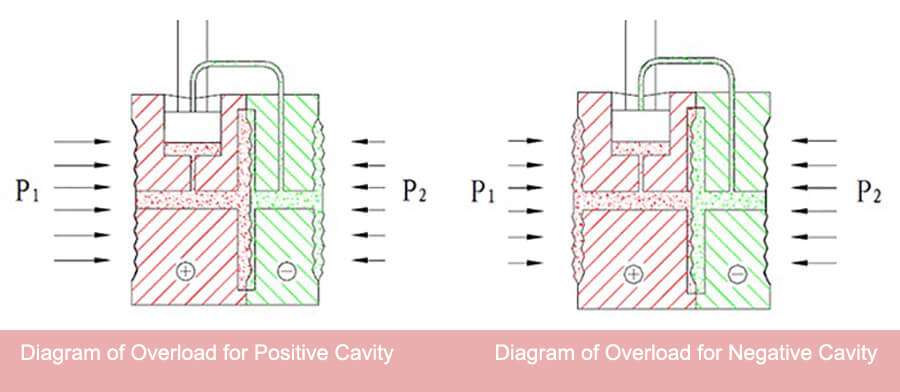
The overload protection design of the EST4300M effectively safeguards the long-term stability of the monocrystalline silicon chip, particularly in scenarios where water hammer phenomena are present.
This is because the rapid pressure surges associated with water hammer can easily damage sensitive components without such protection.
2.2 Superior Rangeability
The EST4300M benefits from the large output signal of the monocrystalline silicon chip. With a 5V constant voltage source excitation, the typical range output reaches 100mV. This makes it relatively easy for the backend electronics and software to implement signal compensation and amplification.
Compared to metal capacitive pressure and differential pressure transmitters, monocrystalline silicon-based pressure and differential pressure transmitters exhibit superior rangeability.
The common pressure transmitters have an adjustable range ratio of up to 100:1, and the micropressure transmitters have an adjustable range ratio of 10:1.
Even after the range is compressed, they still maintain high base accuracy, significantly expanding the adjustable range of the monocrystalline silicon pressure transmitter, which is convenient and meaningful for users.
As shown in Table 1, the accuracy assessment results of three sampled EST4300M differential pressure transmitters after range compression of 10:1 and 100:1 are as follows.
With a full scale of 0-250kPa, after 10 times compression, the range changes to 0-25kPa, and after 100 times compression, the range changes to 0-2.5kPa.
The experimental results show that when the range is compressed by 10 times, the basic errors are 0.019%, 0.012%, and 0.025%, respectively, which can still maintain the accuracy of class 0.05. After compressing the range by 100 times, the basic errors are 0.147%, 0.219%, and 0.197%, respectively, which are still better than the accuracy of class 0.25.
These results demonstrate the EST4300M’s ability to maintain high accuracy even when its measurement range is significantly reduced.
This rangeability is advantageous in applications where a wide variety of pressures need to be measured with the same device or when fine-tuning is necessary to achieve the best possible measurement resolution within a smaller subset of the sensor’s full scale.
This flexibility in the range also implies that fewer models of the transmitter need to be stocked, as one model can cover a wide range of pressures, reducing inventory costs and simplifying process design and maintenance.
| Accuracy | EST4300M-S1 | EST4300M-S2 | EST4300M-S3 |
| 10:1 | 100:1 | 10:1 | 100:1 | 10:1 | 100:1 |
| % | % | % | % | % | % |
1 | Total Error | 0.091 | 0.147 | 0.012 | 0.219 | 0.025 | 0.197 |
2 | Non-Linearity | 0.004 | 0.101 | 0.003 | 0.069 | 0.000 | 0.116 |
3 | Hysteresis | 0.006 | 0.159 | 0.009 | 0.234 | 0.009 | 0.106 |
4 | Repeatability | 0.006 | 0.060 | 0.005 | 0.074 | 0.009 | 0.069 |
| | | | | | | |
Based on your description, Table 2 would present the accuracy assessment results for three EST4300M pressure transmitters after their measurement range has been compressed by factors of 10:1 and 100:1.
Here is a narrative interpretation of what such a table might show, based on the results you mentioned:
The full scale range of the transmitters is 0-40MPa. When the range is compressed by a factor of 10, it changes to 0-4MPa, and when compressed by a factor of 100, it changes to 0-400kPa. The experimental results indicate the following:
- After a 10:1 range compression, the basic errors for the three samples are 0.041%, 0.047%, and 0.034%, respectively. These values demonstrate that the transmitters can still achieve the accuracy of Grade 0.05.
- After a 100:1 range compression, the basic errors for the three samples are 0.15%, 0.063%, and 0.153%, respectively. Despite the significant reduction in range, these errors are still better than the accuracy of Grade 0.25.
The results from Table 2 would suggest that the EST4300M pressure transmitters exhibit excellent rangeability while maintaining high accuracy, even when the measurement range is greatly compressed.
This level of performance makes these transmitters suitable for a variety of demanding applications where precision is critical, even at significantly reduced measurement ranges.
The EST4300M’s ability to maintain accuracy across such a wide range of conditions underscores its flexibility and the robustness of its design, which can be particularly advantageous for users with diverse and variable pressure measurement needs.
| Accuracy | EST4300M-S3 | EST4300M-S4 | EST4300M-S5 |
| 10:1 | 100:1 | 10:1 | 100:1 | 10:1 | 100:1 |
| % | % | % | % | % | % |
1 | Total Error | 0.041 | 0.150 | 0.047 | 0.063 | 0.034 | 0.153 |
2 | Non-Linearity | 0.034 | 0.046 | 0.039 | 0.006 | 0.032 | 0.082 |
3 | Hysteresis | 0.006 | 0.094 | 0.009 | 0.069 | 0.012 | 0.094 |
4 | Repeatability | 0.003 | 0.051 | 0.005 | 0.029 | 0.006 | 0.054 |
2.3 Superior Pressure Hysteresis Performance
Pressure hysteresis, also known as the return error characteristic or simply hysteresis, is an important performance metric for pressure and differential pressure transmitters. The magnitude of hysteresis directly affects the measurement accuracy and long-term drift performance of the transmitter.
A typical error curve comparison between monocrystalline silicon sensors and metal capacitive sensors might look something like this, as show in below figure:
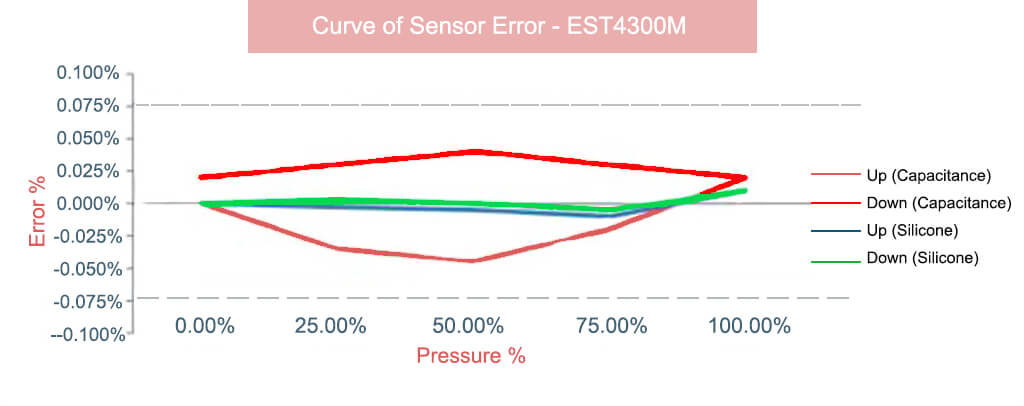
From such a diagram, we would observe that the monocrystalline silicon sensor exhibits a very small hysteresis. The ascending (pressure increasing) and descending (pressure decreasing) curves almost overlap, indicating that the hysteresis is negligible and does not significantly affect the sensor’s output accuracy.
This minimized hysteresis suggests that the sensor’s readings are highly repeatable and reliable, regardless of whether the pressure is increasing or decreasing.
In contrast, the metal capacitive sensor’s error curve would show a larger hysteresis, with the ascending and descending curves forming an open loop. This gap indicates that the sensor’s readings may differ when the pressure is increasing versus when it is decreasing, which could lead to inaccuracies in the transmitter’s output.
The superior hysteresis performance of the EST4300M suggests that it would be particularly suitable for applications where high precision and repeatability are required over a wide range of operating conditions.
The reduced hysteresis would also contribute to the stability of the sensor’s output over time, indicating a design optimized for reliable, long-term use.
2.4 Unique Static Pressure Characteristics
Differential pressure transmitters are commonly used to measure tank levels or pipeline flow rates. If the effect of static pressure is not corrected or compensated for, it can introduce significant errors into the measurement. This effect is particularly pronounced when the level range is small or the relative flow rate is low.
For instance, a capacitive differential pressure transmitter, when used with a flow restriction device to form a differential pressure flow meter, may have a full-scale static pressure error of ≤±2% FS under a working static pressure of 32MPa.
While the zero-point error can be eliminated through zero adjustment, the full-span output error is unavoidable. This static pressure error directly affects flow measurement accuracy and can have a significant impact.
In such applications, the performance of a differential pressure transmitter under static pressure is crucial. If this static pressure error is compensated for, or if the transmitter inherently has minimal static pressure error, the accuracy of the measurements can be greatly improved.
The EST4300M differential pressure transmitter utilizes a unique encapsulation process for its monocrystalline silicon chip. After encapsulation, the inner and outer chambers of the chip reach pressure equilibrium. An illustrative diagram (below figure ) would show the encapsulation of the monocrystalline silicon wafer.
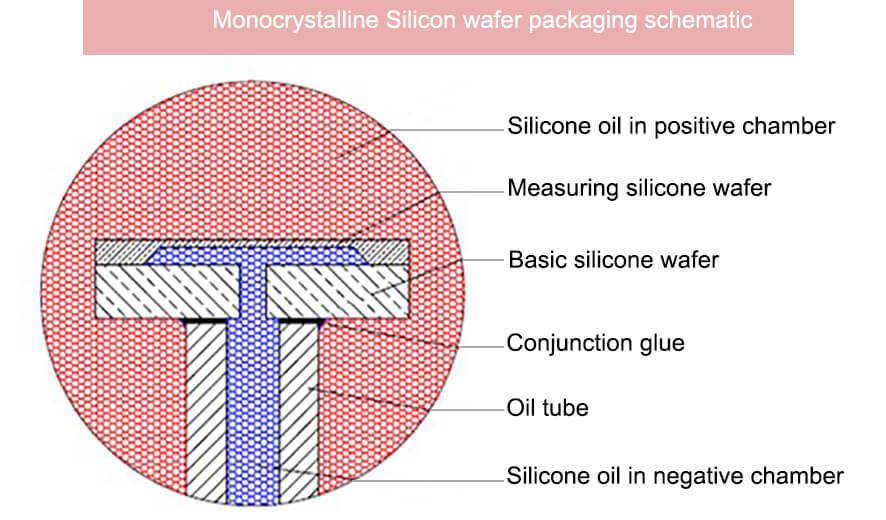
When working static pressure is applied to the positive and negative chambers of the measuring silicon wafer, the pressure is balanced through the silicon oil in the outer positive chamber and the silicon oil in the inner negative chamber, loading the pressure onto the measuring wafer. This process achieves a counterbalance, resulting in minimal bending deformation of the measuring silicon wafer due to the working static pressure.
This approach significantly enhances the static pressure impact performance of the differential pressure transmitter.
By effectively balancing the pressure within the device, the EST4300M is capable of providing accurate measurements even under high static pressure conditions, minimizing errors that could arise from pressure-induced deformation of the sensor element.
This feature makes the EST4300M particularly suitable for high-precision applications in challenging environments where static pressure can have a detrimental effect on measurement accuracy.
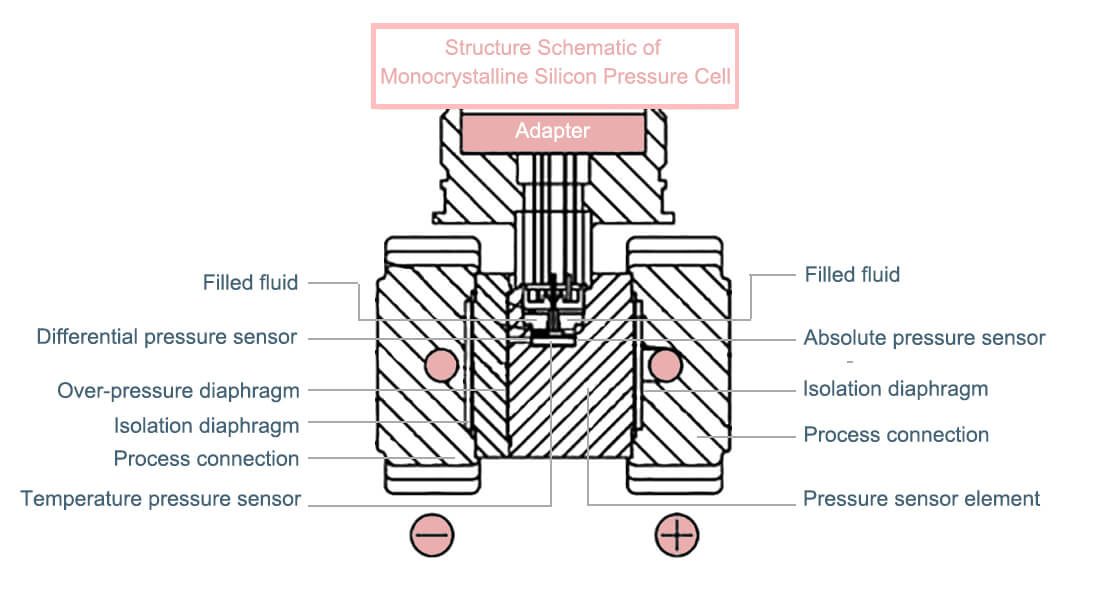
In the application of micro differential pressure transmitters, due to the extremely small magnitude of the differential pressure signal, the sensitivity to the impact of static pressure is very high, and the unique encapsulation design and technology described above cannot completely eliminate or weaken the impact of static pressure.
To address this issue, the EST4300M micro differential pressure transmitter has integrated an absolute pressure sensor within its sensor, which can measure the working static pressure, as shown in the structural schematic of the EST4300M micro differential pressure sensor (refer to above figure).
This absolute pressure sensor can provide real-time feedback of the measured working static pressure signal to the internal microprocessor. The microprocessor uses this static pressure coordinate to automatically correct the micro differential pressure output signal, thus achieving static pressure compensation.
With the unique encapsulation process and the addition of an absolute pressure sensor, the static pressure performance of the EST4300M differential pressure transmitter has been greatly enhanced, thereby ensuring the measurement accuracy and high stability of the pressure transmitter.
2.5 Unique Diaphragm Processing Technology
Compared to the isolation diaphragm welding methods used by American Rosemount’s metal capacitive sensors, Japan’s Yokogawa’s single-crystal silicon sensors, and Europe’s ABB’s silicon differential pressure sensors, the EST4300M differential pressure sensor adopts a more advanced hygienic diaphragm welding method without isolation rings.
This hygienic diaphragm welding technique results in smooth welds with no gaps or dead angles. It allows for direct welding of various diaphragm materials, such as 316L stainless steel, Hastelloy C, tantalum, and Monel.
Click to check more details about Pressure Senor Material
The absence of gaps also permits direct gold plating and PTFE coating on the liquid contact surface. This design and special processing technology significantly extend and expand the range of fluid contact for the differential pressure transmitter and greatly enhance the service life of the transmitter in corrosive environments.
2.6 Unique High-Temperature Remote Transmission Design and Implementation
It is well known that in the application of pressure and differential pressure transmitters, high-temperature remote transmission diaphragm seals pose significant safety risks when the medium temperature exceeds 350°C.
Issues such as silicone oil vaporization, data distortion, or reduced lifespan are likely to occur, which requires the process medium to have a certain working static pressure to form a back pressure ensuring the normal operation of the diaphragm seal. This requirement has limited the application range of remote liquid level measurements by pressure and differential pressure transmitters.
However, the high-temperature remote transmission pressure transmitter designed for temperatures up to 600°C utilizes advanced measurement technology for high-temperature media, with the measurable temperature of the medium reaching up to 600°C.
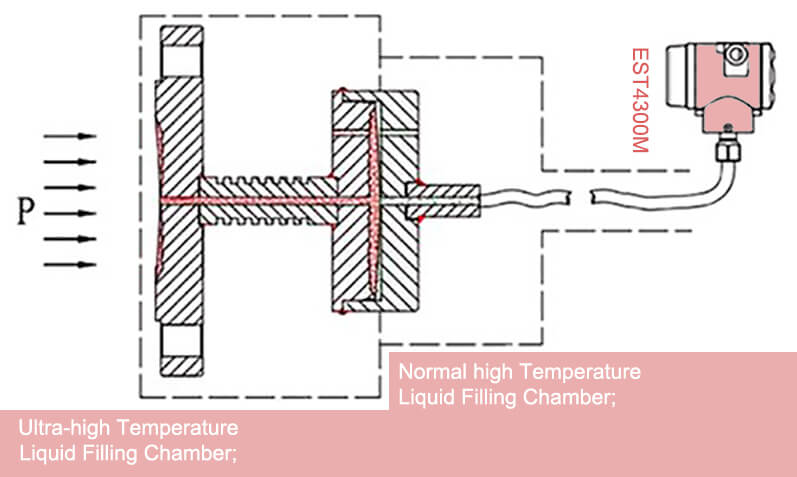
Filled-Fluid Chamber of Super High Temperature
As shown in Figure 8, the schematic diagram illustrates the structure of this ultra-high temperature remote transmission.
This structure is divided into two chambers:
- One for ultra-high temperature filling fluid
- The other for regular high-temperature filling fluid.
A diaphragm, welded between the two chambers, isolates them, and a heat dissipation rod is placed inside the ultra-high temperature chamber. The ultra-high temperature filling fluid, which comes into direct contact with the medium, can withstand temperatures up to 600°C.
However, due to its high viscosity, it is not suitable for filling into capillaries for pressure transmission. Therefore, the pressure is further transmitted through the isolation diaphragm to the chamber with the regular high-temperature filling fluid, ensuring limited pressure transfer and rapid response.
After heat dissipation, the temperature of the heat transferred to the regular high-temperature chamber is significantly reduced, guaranteeing the normal operation of that chamber.
This method expands the application range of high-temperature remote transmitters and improves the reliability and lifespan of ultra-high temperature remote transmitters.
2.7 Performance Indicators and Reliability
Through the technical introduction and analysis of the EST4300M series, we’ve briefly describes the realization process of the EST4300M monocrystalline silicon high-stability pressure and differential pressure transmitter project.
We enhance the overall performance, accuracy, and reliability of high-stability pressure and differential pressure transmitters from multiple aspects: selecting monocrystalline silicon principle chips, stress-free packaging of monocrystalline wafers, eliminating return error, reducing static pressure influence, expanding range ratios, special processing of wetted surfaces, and extending ultra-high temperature measurement capabilities.
Through the introduction and assimilation of various technologies, combined with innovative designs, the EST4300M series of high-stability pressure and differential pressure transmitters has reached the market leadership levels. Its main technical advantages are:
- Accuracy level reaches 0.05, and it has obtained a manufacturing license for measuring instruments, achieving domestic advanced levels.
- The micro-differential pressure transmitter adopts a unique dual overload protection diaphragm technology, achieving a high measurement accuracy of ±0.075%, with a maximum working static pressure of 16MPa and a minimum measurable differential pressure range from -50Pa to 50Pa, far exceeding domestic and international technological levels.
- The highest working static pressure for the differential pressure transmitter can reach 40MPa, with a unidirectional overload pressure of up to 40MPa.
- An absolute pressure sensor can be optionally encapsulated inside the differential pressure sensor for the measurement and display of on-site static pressure, as well as for static pressure compensation, resulting in excellent static pressure performance for the monocrystalline silicon pressure transmitter, with the typical specification static pressure error being an optimal ≤±0.05%/10MPa.
- The integration of the internal absolute pressure sensor also ensures the successful development of the EST4300M multiparameter transmitter, which can be widely used in the field of gas flow measurement and fills the domestic gap in high-end multiparameter transmitters.
- An internal high-sensitivity temperature sensor in the pressure and differential pressure sensors ensures excellent temperature performance, with an optimal ≤±0.04%/10K.
- Low-pressure range gauge/absolute pressure transmitters of 6kPa and 40kPa can use unique non-pressure-loss overload protection diaphragm technology, with a unidirectional overpressure of up to 7MPa, significantly expanding the application scope of low-pressure sensors in special fields.
- The typical long-term zero-point drift is ≤±0.1%/3 years, and it has passed 120,000 cycles of 90% full-scale extreme pressure fatigue testing, achieving a maintenance-free capacity of 10 years.
- It realizes an extremely wide measurement range of 0-100Pa to 60MPa, with the highest 100:1 adjustable range ratio output.
- The remote transmitter uses ultra-high temperature technology, suitable for over 400°C ultra-high temperature measurement situations, breaking through the bottleneck of remote product application and measurement.
Warp up:
Monocrystalline Silicon Pressure Transmitters are vast in their application and highly efficient, with a range of features that make them stand out in the field of pressure measurement.
- Precision: They possess incredibly high accuracy, reaching levels up to 0.075% of Span. This equates to maximum accuracy in the detection and transmission of pressure levels, making them ideal for tasks that require meticulous measurements.
- Stability: These transmitters are renowned for long-term stability, with a drift of less than 0.25% per annum. Hence, despite the passage of time, they continue to provide accurate and reliable performance.
- Wide Pressure Range: With the capability to measure pressure from very low to extremely high levels, typically in the range of 0-0.6 kPa to 0-6 MPa, they can be used in a vast range of industrial applications.
- Temperature Endurance: Monocrystalline Silicon Pressure Transmitters excel in various environmental conditions, proven to function efficiently in a temperature range of -40 to 85°C.
- Corrosion Resilience: Their monocrystalline silicon sensor resists corrosion, ensuring a long life span and reliable performance even in harsh, corrosive environments.
- Exceptional Output Resolution: They offer a resolution of output signal typically below 0.01% Span, allowing the accurate detection of even the smallest pressure changes.
- Diverse Output Signals: These pressure transmitters provide different output signals including digital (HART, PROFIBUS PA, Foundation Fieldbus) and analog (4-20mA), making them easily compatible with a range of existing systems.
- Overpressure Protection: they stand out for their high overload resistance of up to 400% of Upper Range Limit, providing safety and preventing the transmitters from damage in cases of pressure surges.
With these features, Monocrystalline Silicon Pressure Transmitters, including ET4300M, can be deployed across numerous industries from petrochemical, food and beverage to bio-medical and HVAC, providing top-notch performance and invaluable data.